Unlocking the Power of Neuroplasticity: The Science of Brain Rewiring and Transformation
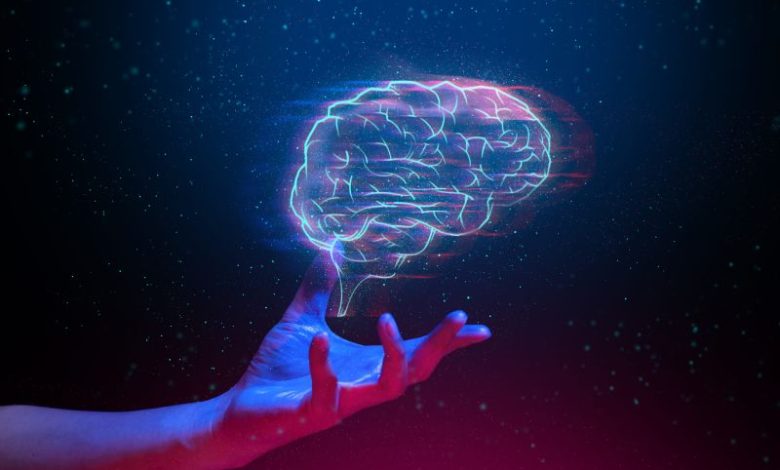
Introduction:
Neuroplasticity, the brain’s remarkable ability to reorganize itself and adapt to new experiences, lies at the heart of human learning, memory, and skill acquisition. From infancy to adulthood, our brains undergo continuous structural and functional changes in response to environmental stimuli, learning opportunities, and behavioral interventions. In this comprehensive exploration, we delve into the intricacies of neuroplasticity, unraveling its mechanisms, implications, and potential for transformative change.
Understanding Neuroplasticity:
Neuroplasticity, also known as brain plasticity, refers to the brain’s capacity to reorganize its neural pathways, synapses, and networks in response to experience, learning, and environmental influences. Contrary to earlier beliefs that the brain’s structure and function were fixed in adulthood, research has demonstrated that the brain remains dynamic and malleable throughout life, capable of adapting to new challenges, acquiring new skills, and recovering from injury or trauma.
Key Mechanisms of Neuroplasticity:
Synaptic Plasticity:
Synaptic plasticity refers to the ability of synapses, the junctions between neurons, to strengthen or weaken in response to activity. Long-term potentiation (LTP) and long-term depression (LTD) are two forms of synaptic plasticity that underlie learning and memory formation. Through repeated activation of neural circuits, synapses become more efficient at transmitting signals, facilitating the encoding and retrieval of information.
Structural Plasticity:
Structural plasticity involves changes in the physical structure of neurons, including dendritic branching, axonal sprouting, and synaptic remodeling. Neuronal growth, pruning, and reorganization occur in response to learning, experience, and environmental stimuli. Structural changes enable the formation of new neural connections, the refinement of existing circuits, and the integration of sensory, motor, and cognitive information.
Functional Plasticity:
Functional plasticity refers to the brain’s ability to redistribute functions across different regions or networks in response to injury, disease, or developmental changes. Following damage to a specific brain area, neighboring regions may assume compensatory functions, enabling individuals to regain lost abilities or adapt to new challenges. Functional reorganization also occurs during skill acquisition, as the brain recruits additional resources to support learning and performance.
Implications of Neuroplasticity:
Learning and Memory:
Neuroplasticity underlies the processes of learning and memory formation, enabling individuals to acquire new knowledge, skills, and behaviors. By engaging in repeated practice, exposure to novel experiences, and active engagement with learning tasks, individuals promote synaptic strengthening and neural integration, enhancing their ability to encode, consolidate, and retrieve information.
Recovery and Rehabilitation:
Neuroplasticity plays a crucial role in recovery and rehabilitation following brain injury, stroke, or neurodegenerative disease. Through targeted interventions, such as physical therapy, occupational therapy, and cognitive rehabilitation, individuals harness the brain’s adaptive capacity to restore lost functions, rewire neural circuits, and regain independence. Neurorehabilitation approaches leverage principles of neuroplasticity to promote functional recovery, maximize rehabilitation outcomes, and improve quality of life.
Skill Acquisition and Expertise:
Neuroplasticity shapes the development of expertise and proficiency across diverse domains, from music and sports to language and mathematics. Through deliberate practice, focused attention, and systematic skill training, individuals induce neuroplastic changes that optimize performance, refine motor skills, and enhance cognitive abilities. By capitalizing on the brain’s adaptive potential, individuals can achieve mastery and excellence in their chosen endeavors.
Mental Health and Wellbeing:
Neuroplasticity offers insights into the mechanisms underlying mental health disorders and resilience. Environmental factors, stressors, and life experiences shape neural circuits implicated in mood regulation, emotion processing, and stress response. Psychotherapeutic interventions, such as cognitive-behavioral therapy (CBT) and mindfulness-based practices, leverage neuroplasticity to promote adaptive coping strategies, resilience, and emotional regulation.
Harnessing Neuroplasticity for Transformation:
Lifelong Learning:
Embrace opportunities for lifelong learning and intellectual stimulation to promote neuroplasticity and cognitive vitality. Engage in intellectually challenging activities, explore new hobbies, and pursue continued education to stimulate neural growth, foster cognitive flexibility, and enhance brain health.
Environmental Enrichment:
Create an enriching environment conducive to brain health and neuroplasticity. Surround yourself with diverse stimuli, engage in stimulating conversations, and expose yourself to novel experiences to promote synaptic connectivity, neural integration, and cognitive engagement.
Mindfulness and Meditation:
Incorporate mindfulness practices and meditation into your daily routine to promote neuroplastic changes associated with stress reduction, emotional regulation, and mental well-being. Mindfulness meditation techniques cultivate present-moment awareness, enhance attentional control, and modulate brain activity patterns implicated in stress resilience and emotional regulation.
Physical Exercise:
Regular physical exercise promotes neuroplasticity, brain health, and cognitive function across the lifespan. Aerobic exercise increases cerebral blood flow, stimulates neurogenesis, and enhances synaptic plasticity, leading to improvements in memory, executive function, and mood regulation. Incorporate a variety of physical activities, such as cardio, strength training, and flexibility exercises, to optimize brain health and cognitive resilience.
Conclusion:
Neuroplasticity represents a fundamental property of the brain that enables adaptation, learning, and transformation throughout life. By understanding the mechanisms and implications of neuroplasticity, individuals can harness the brain’s adaptive capacity to promote learning, recovery, and personal growth. Through lifelong learning, environmental enrichment, mindfulness practices, and physical exercise, individuals can optimize brain health, foster cognitive resilience, and unlock the potential for profound transformation and self-discovery. As we continue to unravel the mysteries of neuroplasticity, we gain deeper insights into the remarkable capacity of the human brain to adapt, evolve, and thrive in the face of challenges and opportunities.
Synaptic Plasticity: Unraveling the Dynamic Architecture of Learning and Memory
Introduction:
Synaptic plasticity, the ability of synapses to undergo long-lasting changes in strength and structure, lies at the heart of learning and memory in the brain. From the formation of new memories to the refinement of motor skills, synaptic plasticity shapes the neural circuits underlying cognition, behavior, and adaptation. In this comprehensive exploration, we delve into the intricacies of synaptic plasticity, uncovering its mechanisms, significance, and implications for understanding brain function and human experience.
Understanding Synaptic Plasticity:
Synaptic plasticity refers to the capacity of synapses, the junctions between neurons, to modify their strength and efficacy in response to neural activity. These changes can be transient, occurring over short periods, or long-lasting, persisting for hours, days, or even a lifetime. Synaptic plasticity encompasses both potentiation, the strengthening of synaptic connections, and depression, the weakening of synaptic transmission, contributing to the dynamic modulation of neural circuits underlying learning, memory, and behavior.
Key Mechanisms of Synaptic Plasticity:
Long-Term Potentiation (LTP):
Long-term potentiation is a form of synaptic plasticity characterized by the sustained strengthening of synaptic connections following repeated activation of presynaptic neurons and postsynaptic depolarization. LTP is often induced through high-frequency stimulation of synapses, leading to the activation of NMDA receptors, calcium influx, and subsequent molecular cascades that result in the recruitment of additional AMPA receptors to the postsynaptic membrane. This increase in synaptic strength enhances neurotransmitter release, promotes synaptic efficacy, and facilitates the encoding of new memories and learning experiences.
Long-Term Depression (LTD):
Long-term depression is a complementary form of synaptic plasticity characterized by the persistent weakening of synaptic connections following low-frequency stimulation or prolonged low-level activity. LTD is induced through the activation of different signaling pathways and molecular mechanisms than LTP, often involving the removal or internalization of AMPA receptors from the postsynaptic membrane. This reduction in synaptic strength results in decreased neurotransmitter release, synaptic efficacy, and synaptic pruning, facilitating synaptic competition, and refinement during development and learning processes.
Spike-Timing-Dependent Plasticity (STDP):
Spike-timing-dependent plasticity is a Hebbian learning rule that governs the timing-dependent changes in synaptic strength based on the relative timing of presynaptic and postsynaptic neuronal activity. When presynaptic activity precedes postsynaptic depolarization, LTP is induced, strengthening the synaptic connection. Conversely, when postsynaptic depolarization precedes presynaptic activity, LTD is induced, weakening the synaptic connection. STDP enables synapses to undergo bidirectional modifications in response to temporal correlations in neuronal firing, facilitating associative learning, and memory formation.
Implications of Synaptic Plasticity:
Learning and Memory:
Synaptic plasticity underlies the processes of learning and memory formation, enabling the encoding, consolidation, and retrieval of information in the brain. By modulating the strength and efficacy of synaptic connections, neural circuits undergo structural and functional changes that facilitate the storage and retrieval of memories, skills, and experiences. The induction of LTP and LTD at specific synapses enables the selective strengthening or weakening of connections, allowing for the storage of memories with varying degrees of significance and relevance.
Neurodevelopment and Experience-Dependent Plasticity:
Synaptic plasticity plays a critical role in neurodevelopment and experience-dependent wiring of the brain during critical periods of development. Sensory experiences, environmental stimuli, and learning opportunities sculpt neural circuits through the selective stabilization and elimination of synapses, shaping the functional architecture of the brain. Disruptions in synaptic plasticity during critical periods can have profound consequences for sensory processing, cognitive development, and behavioral adaptation.
Neural Adaptation and Plasticity in Disease:
Dysregulation of synaptic plasticity has been implicated in various neurological and psychiatric disorders, including Alzheimer’s disease, Parkinson’s disease, schizophrenia, and depression. Aberrant synaptic function, impaired LTP, and disrupted synaptic homeostasis contribute to synaptic dysfunction, cognitive decline, and behavioral disturbances observed in these conditions. Understanding the molecular mechanisms underlying synaptic plasticity dysfunction may inform the development of targeted interventions and therapeutic strategies for neurological and psychiatric disorders.
Therapeutic Interventions and Cognitive Enhancement:
Synaptic plasticity represents a promising target for therapeutic interventions aimed at enhancing cognitive function, memory consolidation, and learning capacity. Pharmacological agents, behavioral interventions, and neuromodulatory techniques that modulate synaptic transmission, neurotrophic factors, and molecular signaling pathways have shown potential for enhancing synaptic plasticity and cognitive performance. By harnessing the brain’s adaptive capacity for synaptic plasticity, researchers aim to develop novel approaches for cognitive enhancement and neurorehabilitation in health and disease.
Conclusion:
Synaptic plasticity represents a fundamental mechanism of neural plasticity that underlies learning, memory, and adaptive behavior in the brain. By modulating the strength and efficacy of synaptic connections, neural circuits undergo dynamic changes in response to experience, learning, and environmental stimuli. Understanding the mechanisms and implications of synaptic plasticity offers insights into the fundamental principles of brain function, cognitive development, and neurological disorders. As we continue to unravel the complexities of synaptic plasticity, we gain deeper insights into the dynamic nature of the brain and its capacity for adaptation, learning, and transformation throughout life.
Long-Term Potentiation (LTP): Unraveling the Mysteries of Neural Plasticity and Learning
Introduction:
Long-Term Potentiation (LTP) stands as one of the most significant discoveries in neuroscience, offering profound insights into the mechanisms underlying learning and memory formation in the brain. First described over five decades ago, LTP represents a fundamental form of synaptic plasticity, wherein the strength of synaptic connections between neurons is enhanced following repeated stimulation. In this comprehensive exploration, we delve into the intricacies of LTP, unraveling its mechanisms, significance, and implications for understanding brain function and cognitive processes.
Understanding Long-Term Potentiation (LTP):
Long-Term Potentiation (LTP) refers to the persistent strengthening of synaptic connections between neurons following high-frequency stimulation of presynaptic inputs and postsynaptic depolarization. LTP is characterized by an enduring increase in synaptic efficacy, leading to enhanced neurotransmitter release, greater postsynaptic responsiveness, and a heightened likelihood of action potential generation in the postsynaptic neuron. This sustained enhancement of synaptic transmission is believed to underlie the processes of learning and memory formation in the brain.
Key Mechanisms of Long-Term Potentiation (LTP):
Glutamatergic Neurotransmission:
Glutamate, the primary excitatory neurotransmitter in the brain, plays a central role in mediating LTP at most excitatory synapses. During LTP induction, glutamate released from presynaptic terminals binds to postsynaptic receptors, including NMDA (N-methyl-D-aspartate) receptors and AMPA (α-amino-3-hydroxy-5-methyl-4-isoxazolepropionic acid) receptors, initiating a cascade of molecular events that culminate in synaptic potentiation.
NMDA Receptor Activation:
NMDA receptors serve as molecular coincidence detectors, requiring both glutamate binding and postsynaptic depolarization for activation. During high-frequency stimulation, postsynaptic depolarization removes the magnesium blockade from the NMDA receptors, allowing calcium influx into the postsynaptic neuron. Calcium influx through NMDA receptors triggers intracellular signaling pathways that contribute to the induction and expression of LTP.
Calcium Signaling and Intracellular Cascades:
Calcium ions serve as key mediators of synaptic plasticity, regulating numerous intracellular signaling pathways implicated in LTP induction and expression. Calcium influx through NMDA receptors activates protein kinases, such as calcium/calmodulin-dependent protein kinase II (CaMKII) and protein kinase C (PKC), which phosphorylate downstream targets involved in synaptic potentiation. These include AMPA receptor subunits, synaptic scaffolding proteins, and transcription factors that modulate gene expression and synaptic remodeling.
AMPA Receptor Trafficking:
LTP induction leads to the insertion of additional AMPA receptors into the postsynaptic membrane, increasing the number of receptor channels and enhancing synaptic responsiveness. This process, known as AMPA receptor trafficking, contributes to the expression of LTP by strengthening the synaptic response to glutamate release and promoting the recruitment of additional synaptic resources to support enhanced synaptic transmission.
Implications of Long-Term Potentiation (LTP):
Learning and Memory:
Long-Term Potentiation (LTP) is widely regarded as a cellular model of learning and memory, reflecting the enduring strengthening of synaptic connections that underlies memory formation. By facilitating the encoding and storage of information in neural circuits, LTP enables the acquisition of new knowledge, skills, and experiences. The induction of LTP at specific synapses leads to the formation of memory traces or engrams, which represent the neural substrates of memory storage and retrieval.
Neuroplasticity and Brain Development:
Long-Term Potentiation (LTP) plays a crucial role in neuroplasticity and brain development, shaping the architecture of neural circuits during critical periods of synaptic refinement and maturation. Sensory experiences, environmental stimuli, and learning opportunities drive the induction of LTP, promoting synaptic strengthening and circuit consolidation in developing brains. Disruptions in LTP mechanisms during critical periods can have profound consequences for neurodevelopmental disorders and cognitive development.
Synaptic Plasticity and Neural Networks:
Long-Term Potentiation (LTP) contributes to the dynamic remodeling of neural networks, facilitating the selective strengthening of synaptic connections that underlie adaptive behaviors and cognitive functions. Through the induction of LTP, neural circuits undergo structural and functional changes that optimize synaptic efficacy, promote information processing, and support behavioral adaptation. LTP mechanisms govern synaptic plasticity across diverse brain regions, enabling the flexible modulation of neural circuits in response to changing environmental demands.
Therapeutic Interventions and Cognitive Enhancement:
Understanding the mechanisms of Long-Term Potentiation (LTP) has implications for the development of therapeutic interventions aimed at enhancing cognitive function and treating neurological disorders. Pharmacological agents, such as NMDA receptor agonists and modulators, have been investigated for their potential to enhance LTP and cognitive performance. Additionally, behavioral interventions, cognitive training programs, and neuromodulatory techniques may harness LTP mechanisms to promote cognitive enhancement and neurorehabilitation in health and disease.
Conclusion:
Long-Term Potentiation (LTP) represents a fundamental mechanism of synaptic plasticity that underlies learning, memory, and neural adaptation in the brain. By elucidating the molecular and cellular mechanisms of LTP, researchers gain deeper insights into the fundamental principles of brain function, cognitive processes, and neurological disorders. As we continue to unravel the mysteries of LTP, we unlock new avenues for understanding brain plasticity, cognition, and behavior, paving the way for innovative therapeutic strategies and interventions to enhance human health and well-being.
Glutamatergic Neurotransmission: Unveiling the Key to Excitatory Signaling in the Brain
Introduction:
Glutamatergic neurotransmission stands as a cornerstone of brain function, mediating the majority of excitatory signaling in the central nervous system (CNS). Glutamate, the primary excitatory neurotransmitter, plays a pivotal role in a myriad of cognitive processes, including learning, memory, and synaptic plasticity. In this comprehensive exploration, we delve into the intricacies of glutamatergic neurotransmission, unraveling its mechanisms, significance, and implications for understanding brain function and neurological disorders.
Understanding Glutamatergic Neurotransmission:
Glutamatergic neurotransmission refers to the process by which glutamate, the primary excitatory neurotransmitter in the brain, is released from presynaptic terminals, binds to postsynaptic receptors, and mediates excitatory signaling between neurons. Glutamate acts on a diverse array of ionotropic and metabotropic receptors, modulating neuronal excitability, synaptic transmission, and network activity throughout the CNS. Glutamatergic synapses are ubiquitous in the brain, playing a critical role in sensory processing, motor control, and higher cognitive functions.
Key Components of Glutamatergic Neurotransmission:
Glutamate Synthesis and Release:
Glutamate is synthesized from the precursor molecule glutamine by the enzyme glutaminase in presynaptic neurons. Following synthesis, glutamate is loaded into synaptic vesicles by vesicular glutamate transporters (VGLUTs) and stored until released in response to action potentials. Upon depolarization, calcium influx triggers the fusion of synaptic vesicles with the presynaptic membrane, leading to the exocytotic release of glutamate into the synaptic cleft.
Glutamate Receptors:
Glutamate exerts its effects by binding to and activating two main classes of receptors: ionotropic glutamate receptors and metabotropic glutamate receptors. Ionotropic glutamate receptors, including NMDA (N-methyl-D-aspartate) receptors, AMPA (α-amino-3-hydroxy-5-methyl-4-isoxazolepropionic acid) receptors, and kainate receptors, are ligand-gated ion channels that mediate fast excitatory neurotransmission. Metabotropic glutamate receptors, on the other hand, are G protein-coupled receptors that modulate synaptic transmission via intracellular signaling cascades.
Excitatory Synaptic Transmission:
Upon binding to postsynaptic glutamate receptors, glutamate mediated excitatory synaptic transmission by inducing depolarization of the postsynaptic membrane. Activation of AMPA receptors leads to the influx of sodium ions and depolarization of the postsynaptic neuron, resulting in the generation of excitatory postsynaptic potentials (EPSPs). NMDA receptors, which are permeable to both sodium and calcium ions, play a crucial role in synaptic plasticity and memory formation.
Glutamate Clearance:
Following neurotransmission, glutamate is rapidly cleared from the synaptic cleft to terminate signaling and prevent excitotoxicity. Glutamate transporters, located on both presynaptic terminals and neighboring glial cells, actively remove glutamate from the extracellular space and transport it back into cells for recycling or metabolism. Dysregulation of glutamate clearance mechanisms can lead to excessive glutamate accumulation, neuronal hyperexcitability, and neurotoxicity implicated in various neurological disorders.
Implications of Glutamatergic Neurotransmission:
Learning and Memory:
Glutamatergic neurotransmission plays a fundamental role in learning and memory processes, underpinning synaptic plasticity, synaptic strengthening, and memory formation. Excitatory synaptic transmission mediated by glutamate receptors, particularly NMDA receptors, is essential for the induction and expression of long-term potentiation (LTP), a cellular model of learning and memory. By modulating glutamatergic signaling, individuals can encode, consolidate, and retrieve information stored in neural circuits, facilitating learning and memory formation.
Synaptic Plasticity and Neural Adaptation:
Glutamatergic neurotransmission is intimately linked to synaptic plasticity, the ability of synapses to undergo long-lasting changes in strength and efficacy in response to activity. Glutamate receptors, particularly NMDA receptors, play a central role in synaptic plasticity mechanisms, including LTP and long-term depression (LTD). By modulating glutamatergic transmission, neural circuits undergo dynamic remodeling, facilitating adaptive behaviors, and cognitive functions.
Neurological Disorders and Disease:
Dysregulation of glutamatergic neurotransmission has been implicated in a wide range of neurological disorders and psychiatric conditions, including Alzheimer’s disease, Parkinson’s disease, epilepsy, schizophrenia, and depression. Excessive glutamate release, impaired glutamate clearance, or alterations in glutamate receptor function can lead to neuronal hyperexcitability, excitotoxicity, and neurodegeneration. Targeting glutamatergic signaling pathways represents a promising therapeutic approach for treating neurological disorders and neurodegenerative diseases.
Therapeutic Interventions:
Pharmacological agents that modulate glutamatergic neurotransmission have shown promise as therapeutic interventions for various neurological and psychiatric disorders. NMDA receptor antagonists, such as ketamine and memantine, have been investigated for their potential to alleviate symptoms of depression, schizophrenia, and chronic pain. Additionally, glutamate receptor modulators, such as AMPA receptor agonists and metabotropic glutamate receptor antagonists, are being explored as potential treatments for cognitive deficits associated with neurodegenerative diseases and neuropsychiatric disorders.
Conclusion:
Glutamatergic neurotransmission represents a fundamental mechanism of excitatory signaling in the brain, governing synaptic transmission, synaptic plasticity, and neural network activity. By elucidating the mechanisms and implications of glutamatergic neurotransmission, researchers gain deeper insights into the fundamental principles of brain function, cognitive processes, and neurological disorders. As we continue to unravel the mysteries of glutamate signaling, we unlock new avenues for understanding brain function, developing therapeutic interventions, and improving human health and well-being.